To study cells in a more physiologically relevant environment, scientists are turning increasingly to three-dimensional cultures. In particular, 3D cell culturing makes an ideal environment for drug discovery. Consequently, some scientists see this as an approach to improve the batting average in making new medicines.
These techniques can be used to study specific molecules, such as survivin, which is overexpressed in various cancer tissues. Moreover, survivin inhibits apoptosis, and it’s related to the resistance of chemotherapy. So, Shang-Tian Yang, professor of food science and technology at Ohio State University, and his colleagues developed a fluorescent assay based on 3D cell culture to screen drugs that might downregulate survivin. The scientists engineered breast cancer cells to express green fluorescent protein (EGFP) to indicate activity in the promoter for survivin.
“These cells were cultured in three-dimensional (3D) polymer-based scaffolds on a 40-microbioreactor platform (40-MBR) with real-time monitoring of EGFP signals,” the team wrote. “This study demonstrated the potential application of the novel 3D survivin promoter-EGFP reporter assay for high-throughput screening of chemicals down-regulating survivin as a molecular target for cancer therapy.”
That’s just one example of applying 3D cell culture to drug discovery. In many cases, getting the most from these techniques depends on tools that are readily available.
Building the base
“Historically, the use of cellular assays in drug discovery has been limited to cells grown on planar 2D surfaces,” says Tanya Yankelevich, director of product management at Xylyx Bio. “However, cells cultured under those non-physiological conditions are not representative of cells that reside in the complex tissue microenvironment.” Exploring molecular interactions in a more realistic environment drives the trend toward studying drugs in 3D culture. As Yankelevich says, “There has been a growing interest over the past few years in using 3D assays in drug discovery following an increased body of evidence that those provide many advantages over 2D assays.”
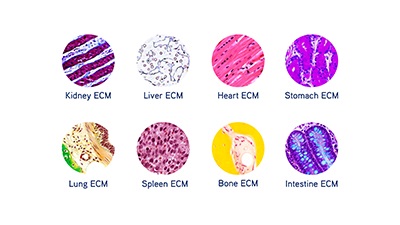
Image: The extracellular matrix creates a scaffold for cell growth, and it is tissue-specific. Image courtesy of Xylyx Bio.
To grow cells in 3D, though, they need a foundation. In natural situations, the extracellular matrix (ECM) creates a scaffold for cell growth. “The cellular microenvironment is critical to successful cell culture, but is often overlooked,” says John O’Neill, CSO at Xylyx Bio. “Commonly used substrates are synthetic, single biochemical components, or derived from a diseased murine source.” That, he points out, creates limited culture conditions. These limitations “underlie the lack of sufficiently predictive models in pharmaceutical drug development,” he adds.
To address this issue, Xylyx Bio makes TissueSpec ECM Hydrogels and Scaffolds for 3D cell culture. “These substrates recreate native cell environments by providing the full milieu of ECM components—proteins, proteoglycans, glycoproteins, growth factors—in a specific tissue,” O’Neill explains. This makes what O’Neill calls a “cell-specific niche in a dish.” The TissueSpec ECM substrates support cell attachment, viability, and phenotypic maintenance.
Those features create a cell system that works well in pharmaceutical research. As Yankelevich notes, the “TissueSpec ECM Hydrogels for 3D cell culture provide a physiologically relevant microenvironment with predictive value in drug testing and cancer research.” Studies also show the value of those substrates. In a recent collaborative study using the Jacket Lung Cell Model from Cellaria, Yankelevich explains, “we have shown preliminary results for the potential of Xylyx Bio’s TissueSpec Lung ECM Hydrogel as a predictive 3D model of human cancer.”
Protocols with purpose
Beyond having the right tools, scientists need tested protocols to get the most accurate results from 3D cell cultures used in drug discovery. An Innovative Medicine Initiative (IMI) consortium—a public/private partnership with partners in academia, contract research organizations and pharmaceutical companies—called PREDECT was focused, in part, on providing such protocols.
According to Ralph Graeser, senior translational medicine expert at Boehringer Ingelheim Pharma, PREDECT’s goal was “to establish protocols for robust in vitro/ex vivo models for target discovery and validation in oncology.” In describing the results, Graeser says, “We generated and published protocols for 2D/3D mono- and co-cultures as well as tissue slices, and also developed imaging solutions that should help both academia and industry to integrate complex models into their early drug discovery flowchart.”
As Graeser and his colleagues reported, they tested the culture models with tumor cell lines from breast, prostate, and lung cancer. They noted, “This guidance should allow other research groups to repeat and extend the data generated by the PREDECT consortium.”
A model that mimics
In drug discovery for ADMET (absorption, distribution, metabolism, excretion, and toxicity) investigations, a completely 3D model isn’t always necessary. “In the liver, polarized hepatocytes in direct contact with the blood supply and the bile canaliculi are arranged in hepatic plates that are only one to two cells thick,” says Jeannemarie Gaffney, senior manager of manufacturing and process development at BioIVT.
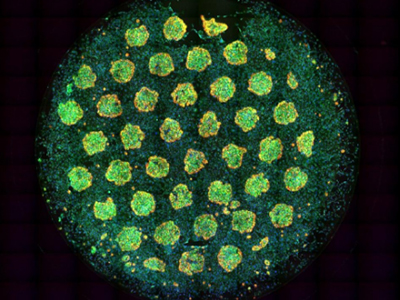
Image: This multicolor image of hepatocyte islands in a 96-well HEPATOPAC® plate shows distinct contrast from the surrounding fibroblasts. Image courtesy of BioIVT.
BioIVT designed its HEPATOPAC® products to model that cellular environment. As Gaffney explains, “The micropatterned design of all HEPATOPAC products simulates microscale in vivo architecture of the liver.” For example, these products produce islands of hepatocytes that form a network of healthy bile canaliculi. This is “indicative of a metabolically active and functional hepatic system,” Gaffney says. “HEPATOPAC products are essentially fully functional hepatic plates contained within the wells of an industry-standard multi-well plate.”
Consequently, this technology can be used to create in vitro liver models. With those models, scientists can study liver metabolism and the effects of toxic substances. Scientists can buy HEPATOPAC cultures created from primary hepatocytes—human, rat, monkey, mouse, or dog—that are surrounded by stromal cells. “The HEPATOPAC configuration stabilizes the hepatic phenotype retaining in vivo functionality in a long-term in vitro culture,” Gaffney says.
These cultures can be used in a range of pharmaceutical studies. As examples, Gaffney mentions a liver-disease model, preclinical toxicity screening, mechanistic/investigative toxicology, metabolite identification, clearance/metabolic stability predictions, transporter-mediated drug uptake and biliary efflux, and drug-drug interactions, including metabolism-mediated and transporter-mediated. “Compared to conventional hepatocyte models, HEPATOPAC shows better in vitro-in vivo correlations, especially for medium- and low-turnover compounds,” Gaffney explains.
In all culture-based applications for drug discovery, scientists seek the most realistic setting. And advances in cell culture promise to improve the odds of finding new medicines.