Nearly 150 therapeutic proteins have been approved by the FDA for sale in the U.S. as of 2018 to treat cancer, autoimmune diseases, and other disorders. Many of these are glycosylated, meaning that they are decorated by branched carbohydrates at one or more amino acid residues. Glycosylation is a complex, sequential process affected by variables such as the host cell’s enzymatic complement and culture conditions, as well as downstream bioprocessing. Thus there is typically a mixture of different carbohydrate structures at different sites (termed macro-heterogeneity), as well as structural variability at any given site (micro-heterogeneity).
A therapeutic’ s carbohydrate makeup can affect its safety, efficacy, and clearance, and so the glycan profile is often considered a critical quality attribute (CQA). Monoclonal antibodies (mAbs)—although their glycosylation profiles are relatively simple compared with many other proteins—are no exception, so their glycan profiles must be monitored, analyzed, and controlled.
Match it
Glycans may affect a molecule’s pharmacodynamics (PD) or its pharmacokinetics (PK), or even its immunogenicity. For an innovator molecule, “it is what it is, so you’re characterizing the molecule so you get an understanding of what’s typical for your production process, and you can replicate that as closely as you can from batch to batch,” says Catriona Thomson, Director, R&D and Technical Services at Sartorius Stedim BioOutsource.” For a biosimilar, on the other hand, “because the innovator will already have a pre-determined glycan profile, you want to try to match that as closely as possible.”
There are several different levels at which a therapeutic protein’s glycoprofile can be determined, each yielding different pieces of information. Releasing the glycans from the protein and separating them by high-performance liquid chromatography (HPLC), for example, is a good way to know which glycoforms are present and how many there are, but tells nothing about where the glycans are attached to the protein. Enzymatically digesting the protein and examining it at the glycopeptide level yields information about attachment sites. And a glycoproteomic approach, looking at either the intact protein or large fragments thereof, is perhaps the best way to determine glycosylation patterns—how the glycans are combined together at which sites on the protein.
Regulators may also want to know the protein:carbohydrate ratio, and the monosaccharide distribution— “how much fucosylation is there, how much sialylation is there, whether the sialic acid is in the glycolyl form or in the acetyl form,” says Biswa Choudhury, the University of California at San Diego’s GlycoAnalytics Core Director. This can be done very simply: “You just hydrolyze that glycoprotein, run it on an instrument called HPAC-PAD [high performance anion exchange chromatography with pulsed amperometric detection], and then you see the profile.”
For the most part—at least for mAbs— “you don’t need to do it every time, because people are relying mostly on the [released glycan] fingerprint,” he adds.
mAbs
Most mAbs are tetramers consisting of two identical heavy chains and two identical light chains. There is a single, conserved glycosylation site on the constant (Fc) region of the heavy chains, at asparagine 297. About a quarter of mAbs contain another N-linked glycan in the variable antigen binding (Fab) region. No O-linked glycans are expected to be found.
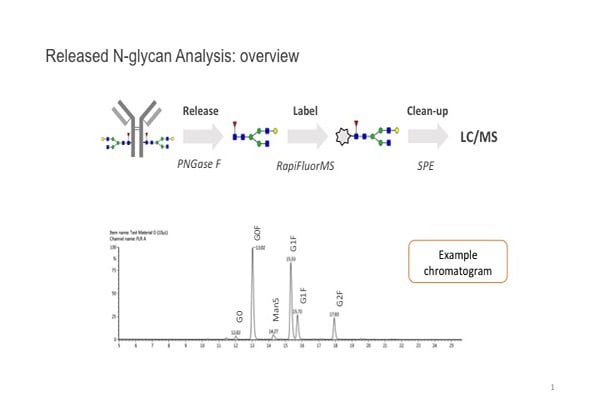
Multiple methods are used to fully determine the structural characteristics of a mAb, but for practical purposes, “the released glycan gives you the greatest sensitivity and the most accurate quantitation. So for us it’s the gold standard approach to accurately assessing the profile of the different glycans,” notes Martin De Cecco, Senior Scientist, Technical Development, at Sartorius Stedim BioOutsource.
The PNGase F enzyme is typically used to remove N-linked glycans from the protein backbone. These are then tagged with a fluorescent label to allow it to be detected. “Glycans are also very polar, very hydrophilic, and derivatizing them improves our ability to separate them as well,” De Cecco explains. They are cleaned up and separated, usually by hydrophobic interaction chromatography [HILIC], and visualized with a fluorescence detector.
Sometimes it’s difficult to distinguish glycans on the basis of retention time alone. “We also have the option of doing MS [mass spectrometry] analysis—we have online MS,” he continues. “So from a characterization point of view, having the mass information really helps us to discriminate between different glycans.”
Generally, “for well-characterized glycan structures, after you do the identification using MS and are able to assign the chromatography peaks, then next time you don’t have to use MS under the same separation method,” points out Xiangkun Yang, a scientist at Regeneron.
How glycans matter
Yang points out that glycosylation is a post-translational modification (PTM) involved in host immune recognition, lysosomal trafficking, and protein folding. For protein therapeutics such as mAbs it is known to enhance protein stability, and reduce immunogenicity by inhibiting protein aggregation and hiding potentially immunogenic epitopes.
We leave off with some more specific examples of how the glycan profile may be an important factor in biopharmaceuticals.
Glycans can modify the function of the molecule: for example, higher levels of core fucose reduce binding of the antibody to the CD16a receptor on the surface of effector cells, such as natural killer cells, that are responsible for antibody dependent cellular cytotoxicity (ADCC). “So less core fucose results in higher ADCC activity,” explains Thomson. This would make fucose content a CQA for an antibody drug like Rituxan or its biosimilars whose mode of action (MOA) is to kill the cells bearing its cognate antigen. Conversely, the MOA of mAbs like Avastin is to bind to and block its cognate antigen. “You don’t want Avastin to have ADCC activity, … and if you wanted to make a biosimilar to Avastin you would have to demonstrate to the regulators that there hasn’t inadvertently been some ADCC activity introduced.”
Different host cell lines produce different types of glycans. “Specifically, I’m thinking of an α-gal structure. … The other is a type of sialic acid called NGNA for short. Those are potentially immunogenic. To my knowledge they are not expected to be produced by CHO [Chinese hamster ovary cells, in which most mAbs are produced], but they can be produced by other cell lines,” says De Cecco. “We know that there are protein therapeutics on the market that have these types of sugar on them at low levels. So it’s a case of balancing the risk.”
The more high-mannose on a protein, the more quickly it can be removed from circulation by mannose receptors in the liver, Yang notes. Conversely, the liver contains receptors for asialo-glycoproteins, meaning that the presence of sialic acid tends to increase a glycoprotein’s half-life.
Partnering to Accelerate Glycomics Research
In June, New England Biolabs (NEB), Waters, and Genos announced they would work together to advance The Human Glycome Project, a global initiative to map the structure and function of human glycans.
The Human Glycome Project is planning to analyze tens of thousands of samples over the next three years. The biggest technical challenge, according to Chris Taron, Scientific Director of Protein Modification Research at NEB, will be the high-throughput aspect of this endeavor, and that is where the partners come in. NEB will be supplying a version of its Rapid PNGase F technology that is compatible with the Waters GlycoWorks N-glycan sample preparation kit. Rapid PNGase F completely deglycosylates monoclonal antibodies and serum IgG in just 10 minutes. “[T]he increased speed of sample preparation these technologies permit will dramatically improve process throughput,” Taron says.
Glycans are structurally variable metabolites whose composition is affected by the coordinated action of hundreds of gene products in complex biosynthetic pathways. “As such, they are the end-recipients of much cellular information,” explains Taron. “As cellular gene expression patterns change due to normal events (e.g., aging, adolescence, menopause, etc.) or disease onset (e.g., cancers) these changes affect the composition of the glycome. Such changes are not only potential biomarkers, but also give insights into the functional effectors of different pathophysiological processes. The hope is that glycans, either on their own, or in combination with other classes of biomarker (e.g., DNA, RNA, proteins, lipids), may give a more holistic view of cellular function, disease progression, or treatment efficacy.
“Thus, as we learn more about the function of the human glycome in the context of clinical science, this information is likely to translate to new diagnostic and therapeutic strategies,” Taron adds.
N-glycan assay workflow image courtesy of Sartorius.