Every experiment needs controls to assure that the results actually mean what they claim. This is especially true in highly multiplexed flow cytometry, where not only do experimental samples need to be compared with normal, untreated cells, but the processes themselves—from sample prep and choosing the appropriate reagents through instrumental setup and operation—can be confounding variables.
In this article, we share some expert advice on everything from how to control for spreading error to what isotype controls are (and are not) good for.
Calibration controls
Say you’re a researcher running samples in a flow cytometry core facility. You assume that the instrument is operating the way it’s supposed to, that it’s properly calibrated, the detectors are linear and in the sweet spot, that the lasers are aligned correctly, and the like. “For most people they’re just showing up at the facility and all that doesn’t need to be visible to them,” says Kelly Lundsten, business segment manager, advanced cytometry, at BioLegend. “That’s why core directors are paid.”
The University of Rochester Medical Center shared resources laboratories performs quality control on their flow cytometers at 7:00 every morning. And even though the machines are very robust, it’s possible for them to go out of kilter during the day, points out director Timothy Bushnell. “An individual researcher should also design their own QC for their process, because that gives them peace of mind.”
He recommends they run a set of standards like Spherotech’s 6-peak Rainbow beads and have a template with a target value, “so when they’re checking their voltages on the PMT [photomultiplier tube] they’re making sure they’re getting at that target plus or minus some percentage.” Seeing a significant voltage jump from one day to the next may indicate a dirty flow cell or a problem with the laser, for example. “Rather than waste your samples and lose data, you can go and say, ‘I think there’s something wrong with the instrument.’”
Compensation controls
Fluorochromes don’t excite or emit at only a single wavelength, but rather across a spectrum with varying intensities that may overlap with those of others. Thus in a multi-color experiment it’s important to determine and correct spillover over into non-intended channels. To do so, samples (either cells or antibody capture beads) are stained with a single fluorophore (for example, FITC) and its contribution to another channel (such as APC) is compensated so that the APC signal is negative. The process is repeated for each fluor and channel.
Aja Rieger, flow cytometry core manager, at the University of Alberta, relates four “essential rules” for creating compensation controls:
- First, because fluorophores in the same channel may have different spectra, it’s important to use an exact match. “For instance, FITC cannot be used as a compensation control for GFP.” And due to differences in tandem chemistry, “the tandem conjugated antibody in the experiment should be the same one (from the same tube) used for compensation.”
- Controls must be at least as bright as the experimental samples. Otherwise “you’ll have under-compensated values in your experimental samples.”
- Autofluorescence for negative and positive populations should be the same for any given control.
- And due to the stochastic nature of fluorescence, it’s imperative to collect enough events—at least 5,000—to allow for an accurate calculation of the mean fluorescence intensity.
Process and biological controls
There does not seem to be a universal lexicon of flow cytometry control terminology—for example, what is a negative or a positive control? an experimental control? a process control? a biological control? or an assay control? Nonetheless, some principles are generally agreed to be important in order to tease out changes genuinely due to the experimental intervention itself, rather than having arisen as an artefact of the experimental process.
Take the negative control. Unstained cells allow the researcher to control for background autofluorescence that may be inherent in the particular cell type. Negative cells may be untreated samples, to contrast with the experimental samples. But “negative control” can also refer to cells expected not to express the antigen of interest. And because activation may affect more than just the antigen marker being tested for—it may increase autofluorescence, or down- or up-regulate other antigens, notes Lundsten—it’s thus imperative to know what variable is being controlled for, and match the controls to the samples and handle them otherwise identically.
Antibodies of the same isotype but different specificity, once a go-to negative control, are now considered only to control for adequate washing and blocking. They may be useful in certain limited cases—such as with B cells, where the cells’ Fc receptor is likely to bind to the antibody non-specifically, or where the fluor itself may be attracted to a surface protein. But isotype controls “shouldn’t be used to subtract the background from your signal,” emphasizes Rieger.
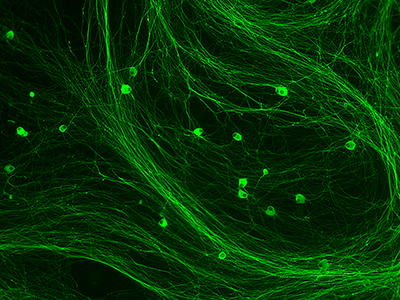
Dead cells are inherently sticky and may non-specifically bind labeled antibodies, and scatter is not always sufficient to gate them out. Controlling for dead cells by incorporating a viability dye—typically a DNA stain like DAPI or 7AAD or DRAQ5—to allow discrimination of live from dead cells “can really help clear up the difference between true positive and true negative staining in a live sample,” notes Wayne Austin, product development scientist at Abcam.
Image: Cultured rat dorsal root ganglion cells stained with rabbit monoclonal anti-beta III Tubulin antibody (ab52623) at 10x magnification. Image courtesy of Abcam.
Gating controls
Single-color controls indicate spillover into other channels. But “once you pass six to eight colors, you can’t use your single-color biological controls to gate your cell populations anymore,” notes Lundsten. Fluorescence-minus-one (FMO) controls—which contain all the fluors but the one being measured—gauge the spillover of those other fluors into the channel of interest. This, in turn, allows more accurate gates to be drawn.
Austin considers FMOs largely a relic of older compensation methods—overly complex and generally unnecessary given today’s instrumentation and software. But he does allow that FMOs may be useful when dealing with emergent or rare markers, where there is a need for more sensitivity and quantitation.
Others, like Rieger, are more likely to “tell people to run the FMOs for everything the first time they do an experiment, and then look at their data to determine which ones need FMOs and which ones may not.”
Reference and longitudinal controls
One of the many more controls that deserves mention is the reference control. This is a sample with known behavior in the assay that “allows you to know how your assay is performing—if there’s a problem with that control, don’t waste your cells, and figure out what’s going on,” says Bushnell. “It may be a harbinger that there’s a problem with the antibody, or the instrument, but you really need to invest that extra ten minutes to run those type of controls.”
The reference control should be from a readily accessible source, such as a defined mouse strain or a large number of frozen PBMCs from a single source. This lets the reference control double as a longitudinal control, allowing different experiments to be compared one to another (but make sure to run an overlap experiment before the sample runs out, cautions Bushnell).
In fact, many controls synergize with and complement each other. The correct answer to the question, “Is that a staining control, a gating control, a biological control, or an experimental control?” may likely be, “Yes.”