Antibody microarrays, also known as antibody arrays, are simple, economical multiplex assays that can be read using instrumentation common to many labs. Although applications of antibody microarrays vary on a study-dependent basis, the value of antibody microarray technology to scientific research is demonstrated by a growing number of publications citing its use. This article provides a recap of the antibody microarray workflow and comments on key developments that are stimulating uptake.
Benefits of antibody microarrays
“Antibody microarrays serve as versatile platforms capable of multiplexing sensitive protein measurements in a variety of throughputs,” explains Shawna Prange, Director of R&D at Grace Bio-Labs. These span formats capable of detecting several thousand proteins simultaneously, which are often used for biomarker discovery during early-stage research, through to more targeted arrays aimed at tracking a specific biological process. “Importantly, by providing screening for multiple analytes in parallel, antibody microarrays can save researchers sample, time, and money,” adds Catherine McAvoy, Commercial Product Manager at Bio-Techne. “Moreover, since most labs are already equipped with the imaging capabilities necessary for detecting typical microarray readouts, there is not usually a requirement to purchase additional equipment.”
The antibody microarray workflow
Processing samples through an antibody microarray workflow is comparable to running a western blot, although the substrate used for antibody immobilization may differ. “While nitrocellulose membranes facilitate physisorption of antibodies, chemically functionalized glass slides are most often used when a covalent linkage is the preferred immobilization,” reports Prange. More recently, these substrates have been complemented by silicon wafers and gold-coated slides that are intended to enhance fluorescent signals, increase sensitivity, and reduce background. Yet, irrespective of substrate type, samples are simply incubated with the antibody microarray before being detected using specialized reagents.
Search Antibodies Search Now Use our Antibody Search Tool to find the right antibody for your research. Filter
by Type, Application, Reactivity, Host, Clonality, Conjugate/Tag, and Isotype.
“Our Protein Profiler Antibody Arrays use an antibody-spotted nitrocellulose membrane for analyte capture,” explains McAvoy. “Then, a cocktail of biotinylated analyte-specific antibodies is added and the captured proteins are visualized using streptavidin-HRP and chemiluminescent detection. Because the data can be read in the same manner as traditional western blots, researchers can quickly see which analytes are up- or down-regulated without needing access to dedicated equipment.” Antibody microarrays based on fluorescent detection often use glass rather than nitrocellulose as the substrate. They can operate in either a single-color or dual-color mode, with the latter approach being shown to reduce systemic error and enhance reproducibility.
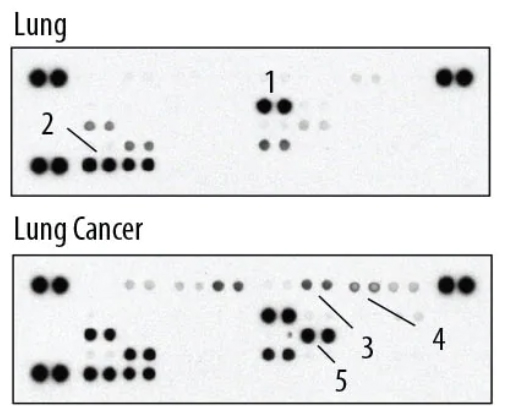
Image: The Proteome Profiler Human Cytokine Array detects 36 human cytokines, chemokines, and acute phase proteins simultaneously. 1) ICAM-1/CD54 2) MIF 3) Complement Component C5/C5a 4) CXCL1/GRO alpha 5) IL-8. Image provided by Bio-Techne.
Scanner considerations for fluorescent detection
While chemiluminescent detection of analytes captured on antibody microarrays can be achieved using either film or digital imaging, fluorescent detection requires a scanner. According to Adriana Lagraulet, Applications Manager at Innopsys, factors to consider when selecting a suitable instrument include the fluorophore characteristics, the microarray substrate, and the dynamic range. “Matching the fluorophore to the excitation and detection capabilities of the scanner is critical to maximize the signal-to-noise ratio,” she says. “The choice of substrate is equally important. For example, to reduce unwanted autofluorescence and increase sensitivity, it is suggested that researchers working with nitrocellulose use near-infrared detection where possible.”
Lagraulet also stresses the significance of the dynamic range, which must be large enough to ensure accurate detection of both rare and abundant targets in the same sample. “Most microarray scanners produce a 16-bit TIF image with a dynamic range of 4-logs and a maximal intensity value of 65535,” she says. “Our InnoScan systems instead allow researchers to generate a 20-bit TIF image with a saturation point of less than 1 million, which represents a 6-log dynamic range. This ensures both weak and strong signals are detected on the same scan, without impacting data distribution.”
Qualitative or quantitative?
All antibody microarrays provide semi-quantitative data based on signal intensity to reveal differences in relative protein expression between samples (e.g., a test sample and a control). However, antibody microarrays can also be configured for quantitative analysis if required. “To be quantitative, an antibody microarray should include a standard curve comprising known concentrations of different analytes of interest,” notes Lagraulet. “The test samples can then be correlated to the standard curve to obtain quantitative protein concentrations.” Usually, quantitative data will only be generated during screening for smaller numbers of targets, and it is common practice to use an orthogonal method (e.g., ELISA) for validating results.
Removing barriers to more widespread adoption
Historically, the complexity of manufacturing microarrays without compromising antibody performance restricted researchers’ use of the technology. But with the development of substrates able to better preserve antibody structure and function, these issues have largely been overcome. Notably, assay reproducibility has also been improved. “Reproducibility challenges have long hindered the widespread adoption of antibody microarrays,” states Prange. “Yet, normalization techniques to account for known variation of replicates, edge effects, spotting effects, and local versus global spatial bias have helped reduce variability and promote greater uptake.”
Numerous publications pay testament to the value that antibody microarrays bring to scientific research. Notably, using antibody microarrays to identify specific protein signatures promises to support the development of more effective treatment options for a broad range of conditions. To illustrate this point, Lagraulet highlights a study published by the CREATE Health Translational Cancer Center at Lund University, where researchers used antibody microarrays to define a signature of 69 serum proteins for differentiating metastatic prostate cancer patients from healthy controls. “Classical serum tests based on measuring total prostate specific antigen (PSA) levels in blood are unable to distinguish between slow-growing tumors and more aggressive forms of prostate cancer,” she says. “In contrast, antibody microarray data has indicated a role for several proteins in metastatic disease, indicating their potential as therapeutic targets.”
Antibody microarrays have also been used within efforts to tackle the ongoing COVID-19 pandemic. “In July of 2020, our Human XL Cytokine Array enabled identification of a biomarker that could accurately discern the severity of COVID-19 and be a possible therapeutic target,” notes McAvoy. “By analyzing the blood of COVID-19 patients, researchers were able to rapidly identify a protein that was consistently elevated in individuals with SARS-CoV-2 pneumonia, particularly those in the intensive care unit, and showed treatment with neutralizing antibodies to suppress the SARS-CoV-2-mediated cytokine storm.”