Conventional cell-based assays typically analyze the average of a population of cells. But sometimes scientists need to single out cells from the rest of the pack. This is especially the case when trying to figure out how cells are responding to drugs or clinical treatments. Sorting of blood cells is used to diagnose various illnesses, including anemia, myeloma, and malaria. The advancement of bioprinting and cell patterning also requires precise cell sorting and manipulation. Although the cell is meant to function as part of something larger than itself, evolving to function within the context of the organ and the body, single cells from the same line or tissue can have diverse genomes, transcriptomes, and epigenomes. When researchers need to isolate a particular cellular subtype or examine cells on an individual basis, they are turning to an array of methods, some modernized versions of older techniques, and others that are just beginning to gain traction.
“Cell separation is indeed a very powerful tool that has greatly contributed to the expansion of biomedical research and clinical applications, and it continues to help push the boundaries of scientific discoveries,” says Miguel A. Tam, senior manager, strategic and product marketing at BioLegend. “Such separations facilitate specific assays to study the influence of certain effectors like cytokines or drugs, with or without the influence of other associated cells in their typical microenvironment,” explains Nitin Kulkarni, field application scientist, MicroMedicine. It also allows for controlled cell manipulation and expansion. And Tam says scientists may want to characterize a defined cell population, verify a cell-specific function, or probe cell-specific signaling pathways. “Whether looking at immune responses, tumor cells, cellular differentiation, or even cell lines, effects that are attributed to the wrong cell type cause biased data,” says Lotta Räty, marketing manager cell separation at Miltenyi. “Cell separation is essentially just a sample preparation step before you start the actual experiment, but it is crucial for conclusive results.”
Kulkarni points out numerous scenarios where separation is required: specific stem cells (SC) such as mesenchymal SCs from bone marrow and CD34+ cells from cord blood for use in regenerative medicine, drug discovery studies, expanding or creating a cell line from a single cell of interest, immune cell isolation for cell therapy, collecting circulating tumor cells to monitor cancer progression, platelet separation to study adhesion and activation, plasma cells for mass antibody production, and many more. He notes that molecular analyses of single cells are also used to gain insight into disease microenvironments. And Räty says that mistakes caused by contamination from other cell types can mean unnecessary cost and loss of time and reagents. “Isolating the relevant cells reduces complexity of the experiment, minimizes background noise, and ensures that smaller effects from rarer cells don’t get lost in the background noise. Therefore the results you gain are statistically relevant.”
Traditional methods get savvier
Cell separation can be either positive or negative. With positive selection, the desired cell type is targeted for removal, usually via an antibody for a surface marker. Negative selection uses antibodies to label unwanted cells for removal leaving behind one cell type untouched.
“Several techniques have been developed to separate cells,” says Tam. “They are based either on the physicochemical properties of the cells or on their phenotype as defined by specific molecules that they express.” These can be divided into adherence, density, or antibody-based methods, but Tam says that separation by adherence is probably the simplest. Explains Kulkarni, “Cell separation and isolation methods are employed either for bulk upstream separation for certain kinds of cells or more downstream to identify and isolate very specific cell types.” He notes that advances in single-cell genomics and proteomics from small sample volumes drives the need for ultrapure isolates without yield tradeoff.
“Ideally, cell separation is fast, highly automated, and most importantly, leaves the cells in their native state so that any effects observed are truly a result of the experiment conditions,” says Räty. But many methods are long, labor intensive, and may lead to cellular changes like activation. This is particularly true for traditional methods that rely on density gradient centrifugation. “For the less specific methods, adherence and density media, reagents haven’t changed much in several years,” notes Tam. Nevertheless, there are still advances. For example, “traditional methods [like density gradients and immunomagnetic techniques] are now evolving to include automation to streamline and optimize the isolation,” says Kulkarni, although he notes that this has not mitigated the need for reagents or centrifugation.
Tam says that the most common antibody-based methods are fluorescence-activated cell sorting (FACS) and magnetic cell sorting. “These methods incur higher costs, but provide the highest versatility for cell separation.” Miltenyi offers a flow cytometry-based MACSQuant® Tyto Cell Sorter, which allows sorting of cells with up to ten parameters in a closed and sterile cartridge environment (MACS stands for magnetic-activated cell sorting). And BioLegend provides a wide selection of reagents, from flow cytometry buffers to antibodies and dyes, including high-performance Brilliant Violet™ and a growing catalog of dyes that are useful in both conventional and spectral flow cytometry.
Magnetic separation kits now available are more versatile. BioLegend’s MojoSort can be used with stand-alone magnets or separation columns and provides both positive and negative cell selection; Tam points out they are also compatible with whole blood isolation. The company’s site suggests “mojo” is in the name because the product will surely yield successful results. These nanobeads are magnetic particles conjugated to antibodies or streptavidin and require a separation buffer and a magnetic separation system.
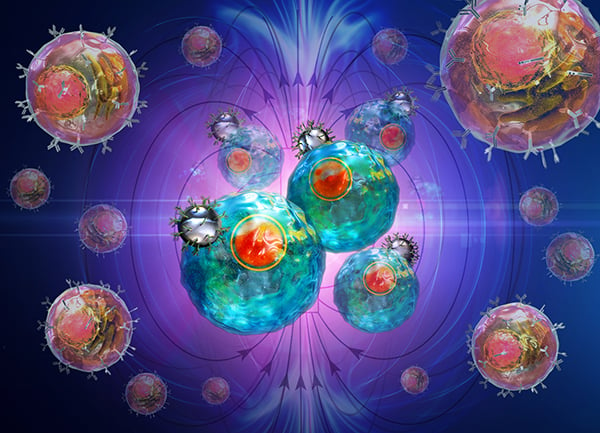
Image: Separation of cells can occur via “Positive Selection” (MojoSort™ Nanobead-bound antibodies directly targeting cells of interest) or “Negative Selection” (targeting unwanted cells). Either way, separated fractions of cells can be used for downstream applications. Image courtesy of BioLegend.
Similarly, Miltenyi offers MACS® Column technology, which Räty says was established 30 years ago, and a broad portfolio of magnetic cell isolation products. These columns use superparamagnetic spheres to amplify the magnetic field, retaining cells labeled with MACS MicroBeads. Räty explains that scientists can use any starting material and expect fast and gentle cell isolation with minimal effect on cells. She notes that recently launched products now allow for the isolation of immune cells directly from blood products without density centrifugation. “We have also made advancements in the field of tumor research, with products that are specifically optimized for complex tumor material to isolate both tumor cells and tumor infiltrating cells. The REAlease MicroBeads can isolate blood or tumor with bead- or label-free cells.”
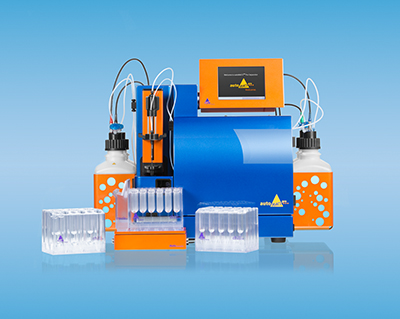
Image: The autoMACS® Pro provides easy, fully automated magnetic cell isolation for a multi-user lab. Image courtesy of Miltenyi.
Newer methods shoot for ease and precision
Isolating cells using microfluidics has recently received more attention given its potential for combining high-throughput with high-precision sorting. And compared to more traditional approaches, microfluidic systems have benefits, such as requiring small amounts of sample and reagents, high sensitivity, miniaturization, and automation, as well as realizing point-of-care testing. Microfluidic sorting involves the application of a force, such as acoustic, electrical, optical, or jet. MicroMedicine recently introduced the Sorterra cell isolation system, which uses inertial-focusing-based automated microfluidic technology to separate and concentrate white blood cells from peripheral whole blood. “There are no density gradients, harsh chemicals, beads, or antibodies,” says Kulkarni, noting that these automated workflows represent key advances in the field, minimizing operator-to-operator variability, increasing consistency, and purity with a run time of only a few minutes.
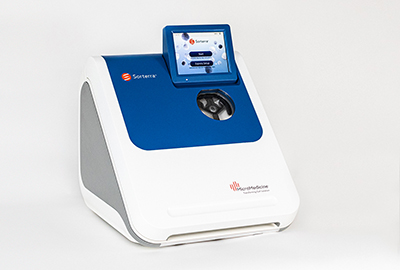
Image: Sorterra™ is an automated microfluidic-based approach for achieving high yield and purity of WBC isolate from peripheral whole blood. Image courtesy of MicroMedicine.
“Novel approaches, such as microbubbles, are constantly being researched and developed as scientists strive to achieve the right balance of manipulation, speed, cost, and specificity and sensitivity of selection,” explains Kulkarni. Antibodies conjugated to microbubbles keep targeted cells in suspension. The idea is to make the desired cells float to the surface where they can be collected. And this can be used in tandem with other methods like MACS and FACS. There is also aptamer-based cell isolation technology. Aptamers (or “chemical antibodies”) are stable DNA or RNA ligands that bind with high affinity and specificity to particular antigens, which include small molecules, peptides, proteins, cells, and tissues. Yet common challenges persist, including sample preparation, cell viability, and balancing purity with yield. Kulkarni points out, “Target cells are a treasured commodity.” And Tam says not to get discouraged. “When in doubt regarding a specific method or technology, do not hesitate to reach out to the vendor or developer,” he says. “Quite often, a simple optimization step is all it takes to separate a successful cell isolation experiment from an experiment you need to repeat.”