The SARS-CoV-2 virus and its resulting disease COVID-19 have greatly impacted livelihoods across the world since its discovery in December of 2019. By late April 2020, the pandemic had infected over 3 million people and claimed over 200,000 lives1. In an unprecedented display of effort and collaboration, the scientific community has made great strides in such a short amount of time. Over 1,000 SARS-CoV-2 genomes had been published by March2. Several crystal structures of key SARS-CoV-2 proteins have now been solved, including the spike glycoprotein, RNA replication machinery proteins, and the two viral proteases3. The life cycle of SARS-CoV-2 is now reasonably well understood, owing to years of study on related coronaviruses. Here we will discuss what researchers have now learned about the viral infection pathway of COVID-19 with an emphasis on the emerging targets for new drugs and vaccines.
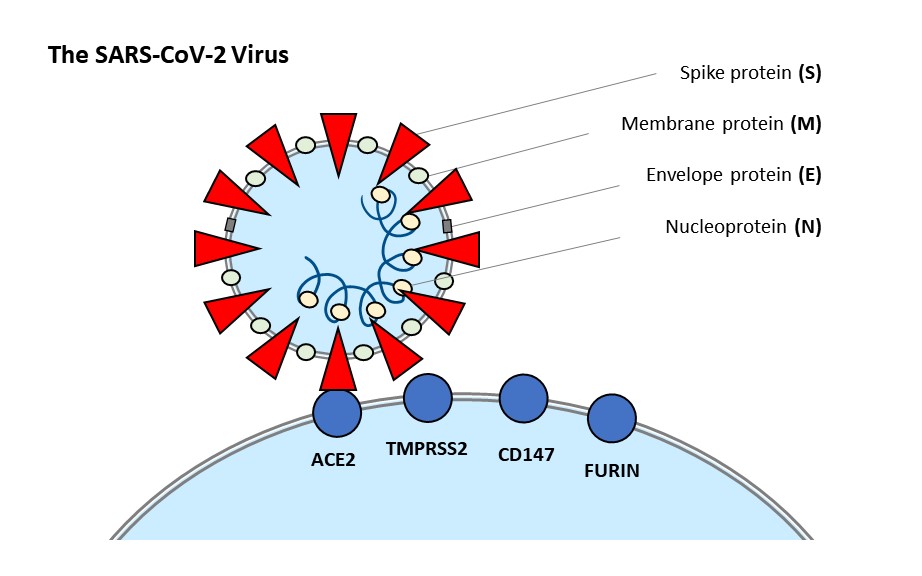
Image 1: This diagram summarizes the components of the SARS-CoV-2 virion and the reported host cell receptors involved in viral binding.
Host cell recognition and binding
As a member of the SARS-related species of coronaviruses, SARS-CoV-2 shares many of its defining characteristics4,5. The spike glycoprotein embedded in the viral lipid envelope recognizes the human host cell receptor, ACE2. An alternative receptor, CD147, has also been reported. After receptor binding, spike is cleaved by certain proteases in order to be primed for membrane fusion with the host cell. The proposed proteases for this task in SARS-CoV-2 are TMPRSS2 and furin6,7.
Discover Coronavirus Research Products
Find, compare, and review reagents
from different suppliers Search
Angiotensin-converting enzyme 2 (ACE2): ACE2 is a cell surface receptor and peptidase that cleaves angiotensin II and other peptide hormones. ACE2 is the known binding partner of the SARS coronavirus spike protein. New functional studies now reveal ACE2 to be the recognized receptor for the entry of SARS-CoV-2 as well. Cell lines stably expressing ACE2 were highly transduced by SARS-CoV-2 pseudoviruses, while treatment with anti-ACE2 antibodies reduced viral entry6,7. These findings make the ACE2-spike interaction an ideal target for antiviral therapeutics.
Related products: ACE2 antibodies, ACE2 ELISA kits, ACE2 proteins, ACE2 vectors, ACE2 inhibitors
Transmembrane serine protease 2 (TMPRSS2): TMPRSS2 is a cell surface protease known to cleave both ACE2 and the spike protein of coronaviruses. Its cleavage of ACE2 is believed to promote viral uptake, while cleavage of spike primes the viral particle for membrane fusion into the host cell. Recent studies have confirmed the TMPRSS2 enzyme activity to be a requirement of SARS-CoV-2 infection of lung cells. Reduced viral entry is observed when cells are treated with camostat mesylate, an inhibitor with clinical applications in other pathways7.
Related products: TMPRSS2 antibodies, TMPRSS2 ELISA kits, TMPRSS2 proteins, TMPRSS2 vectors
Furin: Furin is a protease known for cleaving inactive precursor proteins into their biologically active product. Notably, it functions in cleaving viral envelope proteins including those of HIV, influenza, dengue virus, ebolavirus, and some coronaviruses. While a furin-specific cleavage site has not been found in the SARS coronavirus, a site has been discovered in the protein sequence of the SARS-CoV-2 spike protein. Furin inhibitors have since been proposed in the search for new SARS-CoV-2 therapeutics8.
Related products: Furin antibodies, Furin ELISA kits, Furin proteins, Furin vectors, Furin inhibitors
CD147: Cluster of differentiation 147, also known as Basigin and EMMPRIN, has been implicated as a possible alternative receptor for the SARS-CoV-2 spike protein. An anti-CD147 antibody called meplazumab was shown to inhibit viral infection9. SARS-CoV-2 has been reported to infect T lymphocyte cell lines that express low levels of ACE2, supporting the possibility of CD147 as an alternate entry receptor16.
Related products: CD147 antibodies, CD147 ELISA kits, CD147 proteins, CD147 vectors
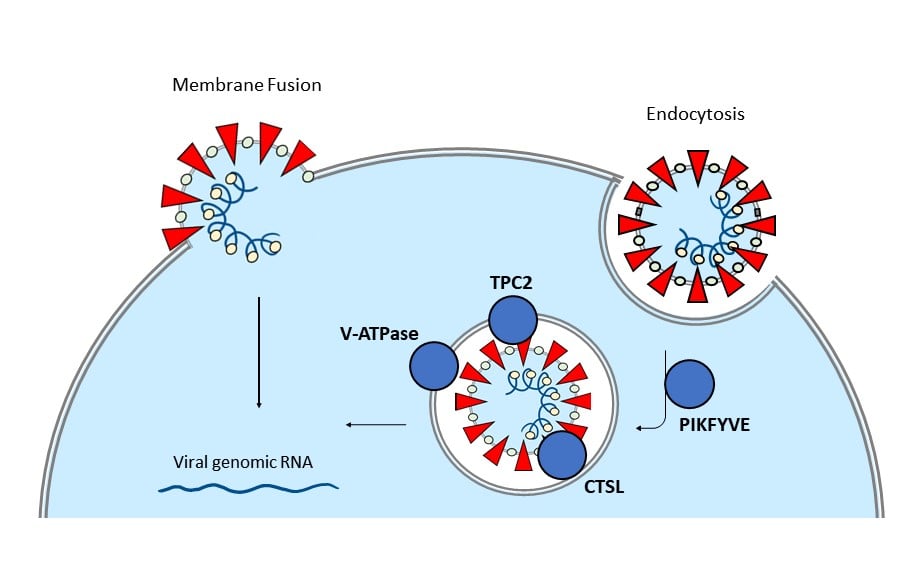
Image 2: The diagram depicts two proposed methods of viral entry (membrane fusion and endocytosis), with a focus on proteins involved in the endocytosis pathway.
Viral entry
In addition to direct fusion with the host cell membrane, SARS-CoV-2 has also been proposed to enter via endocytosis6. This route involves key proteins involved in endosome formation.
Phosphatidylinositol 3-phosphate 5-kinase (PIKFYVE): Endocytosis is an alternate mode of viral entry into host cells and is regulated in part by phosphoinositide lipids. The PIKFYVE kinase synthesizes a class of phosphoinositides that are required in early endosome formation. Activity of PIKFYVE has been discovered to be a requirement in the SARS-CoV-2 infection of human cells via endocytosis. PIKFYVE inhibitors apilimod and YM201636 were found to significantly reduce SARS-CoV-2 entry6.
Related products: PIKFYVE antibodies, PIKFYVE ELISA kits, PIKFYVE proteins, PIKFYVE vectors, PIKFYVE inhibitors
Two pore segment channel 2 (TPC2): TPC2, encoded in humans as TPCN2, is a calcium channel expressed in lysosomal membranes and activated by phosphoinositides. TPC2 has been reported to be essential in the endocytosis of SARS-CoV-2, as its inhibition by tetrandrine led to a decrease in viral entry6.
Related products: TPCN2 antibodies, TPCN2 ELISA kits, TPCN2 proteins, TPCN2 vectors
Cathepsin L (CTSL): Cathepsin L is a lysosomal pH-dependent protease known to mediate the cellular entry of SARS virus via endosomes by priming the virus for membrane fusion10. Studies with broad and specific inhibitors of cathepsin L—E64D and SID 26681509—have shown reduced entry of SARS-CoV-2 pseudovirions6. This suggests a similarly essential function for CTSL in the entry of SARS-CoV-2 and of its potential role as a drug target.
Related products: Cathepsin L antibodies, Cathepsin L ELISA kits, Cathepsin L proteins, Cathepsin L vectors, Cathepsin L inhibitors
Vacuolar-type H+ ATPase (V-ATPase): V-ATPases act as proton pumps that drive protons into endosomes and lysosomes, lowering the pH. An acidic environment is required by pH-dependent proteases such as cathepsins in order to complete the endocytosis of SARS-CoV-2. Bafilomycin A, which specifically inhibits V-ATPase, is a known lysosomotropic agent. Other agents that inhibit endosome acidification include ammonium chloride and chloroquine 6.
Related products: V-ATPase antibodies, V-ATPase ELISA kits, V-ATPase proteins, V-ATPase vectors
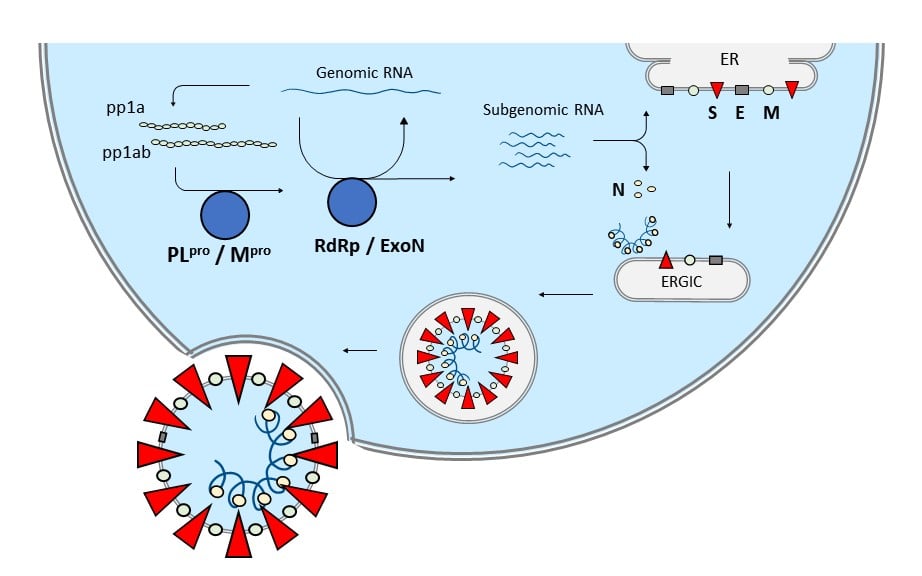
Image 3: This diagram summarizes the replication pathway of new SARS-CoV-2 virions for secretion outside of the host cell.
Viral replication and secretion
Upon uptake of viral genomic RNA, polyproteins and mature non-structural proteins are translated and processed. Assembly of the multi-subunit replicase-transcriptase complex (RTC) leads to both transcription and synthesis of new genomic RNA. Host translation machinery leads to the production of all proteins, with the envelope proteins (M, E, S) being inserted into the endoplasmic reticulum. An endoplasmic reticulum–Golgi intermediate compartment (ERGIC) mediates the assembly and maturation of new viral particles until their final release by exocytosis5.
Papain‐like protease(PLpro): The SARS family of coronaviruses express a papain‐like protease that functions in the proteolytic processing of three nonstructural proteins—Nsp1, Nsp2, and Nsp3. The SARS‐CoV PLpro crystal structure has been solved. Screening chemical libraries for non‐peptidic, reversible inhibitors for PLpro has led to the identification of one lead compound, GRL061711.
Related products: PLpro antibodies, PLpro proteins, PLpro vectors
Main protease (Mpro): Mpro (also known as 3C-like proteinase or 3CLpro) is responsible for processing the remaining nonstructural proteins of SARS coronaviruses, including RdRp and other subunits of the replicase–transcriptase complex (RTC). Several peptidomimetic inhibitor candidates have been reported for SARS‐CoV Mpro, including HCV protease inhibitors telaprevir and boceprevir, and human rhinovirus protease inhibitor rupintrivir11. The crystal structure of SARS-CoV-2 Mpro has recently been reported, along with candidate α-ketoamide inhibitors for blocking its activity12. HIV-1 protease inhibitors lopinavir and ritonavir have been reported to interact with the active site of Mpro 13.
Related products: 3CLpro vectors
RNA-dependent RNA polymerase (RdRp): The RTC of coronaviruses functions in both the replication of the genomic viral RNA and transcription of subgenomic RNA. The complex is formed from multiple nonstructural protein subunits, including RNA-dependent RNA polymerase (nsp12), RNA helicase and 5′-triphosphatase (nsp13), exoribonuclease ExoN (nsp14), and processivity clamps (nsp7 and nsp8)6. The crystal structure of the SARS-CoV-2 RdRp (complexed as nsp12-nsp7-nsp8) has been solved, providing a possible binding and inhibition mechanism for the candidate drug, remdesivir14. Remdesivir is a selective nucleotide analog of ATP that causes the termination of RNA synthesis15.
Related products: RdRP antibodies
Exoribonuclease ExoN: ExoN, or CoV nsp14, is a proofreading 3′–5′ ribonuclease that helps protect against errors in RNA replication, such as those caused by antiviral nucleoside inhibitors. Loss of ExoN proofreading has been found to enhance the antiviral effect of remdesivir17.
Viral immune response
Clinical reports and emerging research now tie SARS-CoV-2 infection to the life-threatening complication known as cytokine storm syndrome18. This extreme form of immune response has been associated with other viruses, including H5N1 and H1N1 influenza virus, variola virus, and SARS-CoV19. Cytokine storms are marked by the uncontrolled elevation of pro-inflammatory cytokines, over-recruitment of immune cells, and systemic inflammation that leads to tissue and organ damage. To account for cytokine storms, a combination therapy approach has been proposed that targets not only the pathogen but also key components of the immune response pathway20.
Cytokines, including chemokines, interleukins, interferons and tumor necrosis factors (TNF), are small secreted proteins that mediate inflammation. Patients with severe COVID-19 requiring intensive care were found to have higher plasma levels of the proinflammatory cytokines GCSF, IP10, MCP1, MIP1A, and TNFα21. Therapeutic targeting of certain cytokines, such as with anti-TNF antibodies, has been proposed as a means of limiting COVID-19 severity22.
Related products: Cytokine ELISA kits, Cytokine antibodies, Cytokine multiplex assays
Table 1: This chart summarizes the various reagents for SARS-CoV-2 research that are listed on Biocompare. Click on an X to view the products.
Target | Antibodies | ELISA Kits | Proteins | Vectors | Inhibitors |
SARS-CoV-2 |
X |
X |
X |
|
|
ACE2 |
X |
X |
X |
X |
X |
TMPRSS2 |
X |
X |
X |
X |
|
CD147 |
X |
X |
X |
X |
|
Furin |
X |
X |
X |
X |
X |
PIKFYVE |
X |
X |
X |
X |
X |
TPC2 |
X |
X |
X |
X |
|
Cathepsin L |
X |
X |
X |
X |
X |
V-ATPase |
X |
X |
X |
X |
|
PLpro |
X |
|
X |
X |
|
Mpro |
|
|
X |
|
|
RdRp |
X |
|
|
|
|
This article has been updated on May 5, 2020.
References:
1. "COVID-19 Map." Johns Hopkins Coronavirus Resource Center, https://coronavirus.jhu.edu/map.html.
2. "Auspice." Nextstrain, https://nextstrain.org/ncov/global.
3. RCSB Protein Data Bank. "RCSB PDB." RCSB PDB: Homepage, www.rcsb.org/news?year=2020&article=5e74d55d2d410731e9944f52&feature=true.
4. Coronaviridae Study Group of the International Committee on Taxonomy of Viruses. “The species Severe acute respiratory syndrome-related coronavirus: classifying 2019-nCoV and naming it SARS-CoV-2.” Nature Microbiology vol. 5,4 (2020): 536–544. doi:10.1038/s41564-020-0695-z
5. Fehr, Anthony R, and Stanley Perlman. “Coronaviruses: an overview of their replication and pathogenesis.” Methods in molecular biology vol. 1282 (2015): 1–23. doi:10.1007/978-1-4939-2438-7_1
expand to see more
6. Ou, Xiuyuan, et al. “Characterization of spike glycoprotein of SARS-CoV-2 on virus entry and its immune cross-reactivity with SARS-CoV.” Nature communications vol. 11,1 1620. 27 Mar. 2020, doi:10.1038/s41467-020-15562-9
7. Hoffmann, Markus, et al. “SARS-CoV-2 Cell Entry Depends on ACE2 and TMPRSS2 and Is Blocked by a Clinically Proven Protease Inhibitor.” Cell vol. 181,2 (2020): 271–280.e8. doi:10.1016/j.cell.2020.02.052
8. Coutard, B., et al. “The spike glycoprotein of the new coronavirus 2019-nCoV contains a furin-like cleavage site absent in CoV of the same clade.” Antiviral research vol. 176 (2020): 104742. doi:10.1016/j.antiviral.2020.104742
9. Wang et al. "SARS-CoV-2 Invades Host Cells Via a Novel Route: CD147-spike Protein." BioRxiv, 14 Mar. 2020, www.biorxiv.org/content/10.1101/2020.03.14.988345v1.
10. Simmons, Graham, et al. “Inhibitors of cathepsin L prevent severe acute respiratory syndrome coronavirus entry.” Proceedings of the National Academy of Sciences vol. 102,33 (2005): 11876–11881. doi:10.1073/pnas.0505577102
11. Hilgenfeld, Rolf. “From SARS to MERS: crystallographic studies on coronaviral proteases enable antiviral drug design.” The FEBS journal vol. 281,18 (2014): 4085–4096. doi:10.1111/febs.12936
12. Zhang, Linlin, et al. “Crystal structure of SARS-CoV-2 main protease provides a basis for design of improved α-ketoamide inhibitors.” Science vol. 368,6489 (2020): 409–412. doi:10.1126/science.abb3405
13. N1utho, Bodee, et al. "Why Are Lopinavir and Ritonavir Effective Against the Newly Emerged Coronavirus 2019? Atomistic Insights into the Inhibitory Mechanisms." Biochemistry, 24 Apr. 2020, pubs.acs.org/doi/10.1021/acs.biochem.0c00160.
14. Gao, Yan, et al. "Structure of the RNA-dependent RNA Polymerase from COVID-19 Virus." Science, 10 Apr. 2020, science.sciencemag.org/content/early/2020/04/09/science.abb7498.
15. Gordon, Calvin J., et al. "Remdesivir is a Direct-acting Antiviral That Inhibits RNA-dependent RNA Polymerase from Severe Acute Respiratory Syndrome Coronavirus 2 with High Potency." Journal of Biological Chemistry, 13 Apr. 2020, www.jbc.org/content/early/2020/04/13/jbc.RA120.013679.abstract.
16. Wang, Xinling, et al. "SARS-CoV-2 infects T lymphocytes through its spike protein-mediated membrane fusion." Nature, 7 Apr. 2020, www.nature.com/articles/s41423-020-0424-9.
17. Agostini, Maria L., et al. “Coronavirus Susceptibility to the Antiviral Remdesivir (GS-5734) Is Mediated by the Viral Polymerase and the Proofreading Exoribonuclease.” mBio vol. 9,2 e00221-18. 6 Mar. 2018, doi:10.1128/mBio.00221-18
18. Wadman, Meredith, et al. “How Does Coronavirus Kill? Clinicians Trace a Ferocious Rampage through the Body, from Brain to Toes.” Science, 22 Apr. 2020, www.sciencemag.org/news/2020/04/how-does-coronavirus-kill-clinicians-trace-ferocious-rampage-through-body-brain-toes#.
19. Tisoncik, Jennifer R et al. “Into the eye of the cytokine storm.” Microbiology and molecular biology reviews : MMBR vol. 76,1 (2012): 16-32. doi:10.1128/MMBR.05015-11
20. D'Elia, Riccardo V et al. “Targeting the "cytokine storm" for therapeutic benefit.” Clinical and vaccine immunology : CVI vol. 20,3 (2013): 319-27. doi:10.1128/CVI.00636-12
21. Huang, Chaolin, et al. “Clinical Features of Patients Infected with 2019 Novel Coronavirus in Wuhan, China.” The Lancet, Elsevier, 24 Jan. 2020, www.sciencedirect.com/science/article/pii/S0140673620301835.
22. Feldmann, Marc, et al. “Trials of Anti-Tumour Necrosis Factor Therapy for COVID-19 Are Urgently Needed.” The Lancet, vol. 395, no. 10234, 9 Apr. 2020, pp. 1407–1409., doi:10.1016/s0140-6736(20)30858-8.