Drug development is a costly endeavor; hence it’s better for drugs to fail fast and early. One of the leading reasons why drugs fail later in the development process, or in the clinic, has to do with translation. The results obtained using biochemical assays, cell-based assays, or animal models often don’t correlate with what is seen when the drug is given to patients. Studies have found that only about one-third of highly cited animal research translates to randomized human trials. The only way to improve this in vitro to in vivo translation is to test compounds very early using models that are more physiologically relevant.
Search Cell culture related products Search Now Search our directory to find the right cell culture tools for your research needs.
“There is a need for human in vitro models with fully tunable characteristics that recapitulate tissue features and are descriptive of the disease state in question,” says Caitlin Hopkins, Senior Doctoral Candidate in Therapeutic Sciences in the Laboratory of Dr. Jeffrey Morgan at Brown University. Some of the strategies to create such physiologically relevant in vitro models involve using appropriate biological materials like human cells, primary cells, stem cells, genetically stable cells, or 3D cells. “These cells must then be grown and maintained in physiologic conditions with extended metabolic capacity and restriction on concentrations,” says Hopkins. “There must be standardization, automation, quality assurance, and validation of procedures to ensure consistency and replicability.”
Designing the right physiological model
Organoids are self-assembled cells with 3D organization, intercellular communication, and recapitulation of developmental processes. Biofabricated organoids are grown on a micropatterned extracellular matrix and have a tunable 3D organization with directed structural perturbations. Spheroids, on the other hand, are circular, free-floating cellular aggregates with low complexity and organization. Both 3D organoids and spheroids have been shown to be physiologically relevant and have been used in diverse applications from disease modeling and drug testing to organ replacement. However, it is evident that the more complex the system, the lower the throughput for its use.
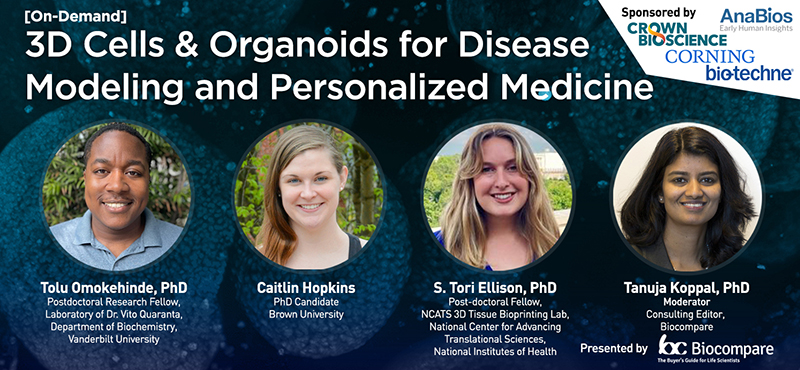
Hopkins is working on developing more predictive 3D models to test pro- and anti-fibrosis stimuli that lead to changes in the fibroblast cells. “Tissue stiffness is a phenotypic hallmark of fibrotic diseases and is often overlooked in some of the contemporary models. We are trying to correlate biochemical alterations with biomechanical changes using our 3D cellular model.” She is creating scaffold-free, self-assembled organoids, sometimes with complex geometries, using a non-adhesive agarose gel. Using this model, she is able to perform many biochemical assays, imaging, histology, PCR, and biomechanical testing on the cells at various time points (7, 14, 21 days) and after treatment with various cytokines and growth factors.
Following the rules and protocols
There are different stages to organoid development and careful procedures and conditions need to be followed to ensure their growth and health. Needless to say, there is a lot of trial and error involved in setting up these 3D models for various applications and assays. According to Hopkins, there are many design considerations that go into creating these organoids so that they are robust and replicable. These include standardizing the mold design and materials, cell density, culture media composition, and use of supplements. Attention to sterility of cells, reagents, and materials is always needed, since the cells are used for long-term studies.
Tolu Omokehinde, Ph.D., a Postdoctoral Research Fellow in the Department of Biochemistry at Vanderbilt University, is working on developing cerebral and lung 3D organoids for studying small cell lung cancer and agrees that there are many considerations that go into growing and maintaining 3D cells. “We use human embryonic stem cells to develop our organoid models, and we find that keeping them as aggregates prevents them from differentiating and helps expand their population,” he explains. “You have to be careful to passage and re-plate the cells as aggregates and not recreate a single-cell suspension.” The cells have to be monitored daily for appropriate morphology changes and certain cell culture reagents and induction media need to be added at specific times to facilitate their growth. “Generation of embryoid bodies is an important step that leads to the differentiation of cells into their lineages. Cells are plated on a low-attachment plates in a specific differentiation media to enable that,” he says. The embryoid bodies then form neural rosette clusters, which eventually generate cerebral organoids and that requires a specific neural induction media as well. Hopkins also uses a number of supplements to stimulate collagen production in her 3D fibrosis models.
Certain aspects of the 3D organoid generation can be very technically challenging. For instance, dislodging the neural rosette clusters to suspend them in media solution involves infusing matrix into the cluster droplet, incubating these delicate clusters for a certain time, and then peeling and rolling them into the differentiation media. But once the cerebral organoids develop, they are quite robust and can continue to grow for many months and can be used for many exciting studies. “We have done some high-content imaging and cell painting to look at individual cellular structures within the organoid to provide insight on how these cells affect the tumor microenvironment,” notes Omokehinde.
Building accuracy and repeatability
S. Tori Ellison, Ph.D., a Post-doctoral Fellow at the National Center for Advancing Translational Sciences (NCATS) 3D Tissue Bioprinting Lab at National Institutes of Health, uses 3D cell culture models to perform high-throughput screening and testing of drug candidates. “For conducting a high-throughput drug screen, you need a functional tissue model that is validated, repeatable, and cheap, along with a disease phenotype that can be controlled and measured, and a marker that can quantify efficacy and toxicity of the drug,” says Ellison.
When testing drug candidates for the treatment of the human simplex virus (HSV), she uses a simple 3D skin model consisting of a dermis layer that has fibroblasts and extracellular matrix, and the epidermis, which has keratinocytes. The top layer of cells is exposed to air, while the bottom layer is exposed to media, which creates an air-liquid interface. Figuring out when and how to layer the cells, where to add the perturbants (top or bottom), how much to use, how many replicates and controls are needed, how to control for background fluorescence, has to be figured out before developing the stable 3D tissue model for high-throughput screening.
“Our study showed that you can mass produce bioprinted 3D skin constructs and they can be quality controlled using histology and barrier function,” says Ellison. “We could infect the constructs in a controlled and repeatable manner and characterize the infection pathway and the cells infected. We were able to perform a high-throughput screen, testing different drugs for their safety and efficacy.” She is now looking to develop 3D skin models for studying psoriasis, atopic dermatitis, squamous cell carcinoma, and innervated models for pain and addiction studies.