Alan Mullan, product specialist for microscopy cameras at Andor, part of Oxford Instruments, is really excited about recent advances in microscopy. This includes new camera technology. “The development of more sensitive, faster, and wider field of view cameras is part of this collective set of technologies that is aiding our understanding across multiple research disciplines,” he says.
This expanding technology intersects beautifully with the unfurling of many life science areas, and the evolution of countless tools, such as the plethora of fluorophores. Indeed, it is a time like no other for science. We visualize and model cellular networks, peek inside of cells, and watch biological phenomena in real time.
And we record as much of it as we possibly can.
Today’s microscope cameras capture worlds previously unseen, make available the hidden, and offer the possibility of sharing these observations with the world. But before that, we need to wade through the countless specifications and features. When striving for the best images possible, where do you begin?
Sensors and sensibility
“The first key is to choose the right optics, which are capable of capturing the phenomena, and then to choose a camera that matches the optics. For example, if an objective’s optical resolution is lower than the targeted structure, then any camera cannot help the observation,” explains Takeo Ogama, senior product and strategy planner at Olympus.
Cameras are often chosen based on the type of sensor housed inside. The two most common are CMOS (complementary metal-oxide semiconductor; or variations thereof) and CCD (charged coupled devices). Both CMOS and CCD sensors detect light in the same way. Both sensors use pixels (a pixel is a single point in a digital graphic image). It is in the conversion of the pixel’s charge to voltage, however, where things differ. (If you’re really itching to read about the physics underlying these sensors, you can start here.) Historically, CCD edged out CMOS every time when it came to sensitivity, noise, and dark current performance (a type of noise related to thermal energy), and CMOS was viewed as the inferior, albeit economic alternative.
After years of development, however, CMOS is flush with its own advantages, including a time-saving sensor read out. According to Mullan, interline CCD sensors have become less popular since the introduction of scientific CMOS (a more sensitive version of traditional CMOS), around 2010. Mullan thinks that sCMOS is replacing CCD as a “general purpose, jack-of-all-trades type of microscope.”
Ogama thinks similarly. “Currently, sCMOS is the most common solution for high-end microscopic imaging. The only disadvantage of sCMOS is the lack of capability for long-time exposure because it causes too much noise on images,” he says.
CCD cameras do still have a place and may be preferred for longer exposures. “They also have some different sensor coatings, which makes them sensitive in the UV and near-IR regions, so we still see interest in them for these niche applications,” explains Mullan.
Then there is also the EMCCD (electron multiplying CCD) for the lowest light conditions. According to Ogama, “EMCCD is extremely sensitive, [and] used to catch fast biological phenomena or bioluminescence.”
Priorities and trade-offs: Finding the sweet spot
Choosing a camera based on the type of sensor is one way to go, but not necessarily the only. Ash Prabala, chief technologist and co-manager at Thorlabs Scientific Imaging, says that there isn’t a way to “intuit” camera technology simply from the imaging modality. “One can’t say for example, if the modality equals fluorescence, then the ideal camera is sCMOS. But one can say, if the light level is low, then the quantum efficiency (QE) and read noise (Nr) are very important.”
QE and Nr are two variables (of several) that determine camera sensitivity. And camera sensitivity is only one of the many features that should be taken into consideration when deciding what camera is needed for the job. He notes that each feature can drive up the cost considerably.
Five key takeaways
- sCMOS cameras are today’s “jack-of-all-trade” cameras. Consider this option when a microscope is used for a variety of techniques at varying light levels. Keep in mind that extremely low light conditions may require an EMCCD camera. Depending on your specifics, there is still a niche market for CCD cameras.
- Camera sensitivity (how well it detects light from a sample) may be the most important parameter for microscopy cameras. Techniques such as confocal or light field microscopy are inherently low light and therefore, a sensitive camera is required. Brightfield microscopy, on the other hand will not require as much sensitivity.
- Sensitivity depends on SNR (signal to noise ratio) and higher is better. To get the best SNR, cameras should have a high quantum efficiency (QE; a measurement of how efficiently photons are translated into electrons) and low noise. Noise is dependent on the type of exposure needed.
- Resolution, the amount of detail that a camera can capture, is dependent on pixel size and field of view. Smaller pixels give a higher spatial resolution at lower magnifications. However, there will be a lower SNR compared to larger pixels (such as those found in EMCCD cameras). The key is to find a pixel size that provides sufficient resolution and good signal to noise. Wider fields now allow scientists to capture more cells or image an entire embryo in one view.
- Speed should depend on the phenomena being captured. Calcium imaging, for example, will require the fastest frame rate possible. The camera must be sensitive enough to handle quick exposures. sCMOS or EMCCD cameras may be suitable choices when speed is a factor.
But Prabala says that by answering the light-level question first, the customer may be guided away from more expensive technologies that might not provide much benefit. “The next question: Are those parameters [QE, Nr, Frame Rate, etc.] so critical that the scientist must spend more than $12 - 15K on a camera with the highest possible QE and the lowest achievable Nr? Or can the desired experimental results be obtained with a Quantalux sCMOS camera that is priced at less than $3K. For this we need to have a figure of merit that ties ‘camera performance’ to ‘How much light do I have to work with?’ That figure of merit is SNR (signal to noise ratio).”
According to Prabala, under very low light conditions, (less than 10 photons/pixel) and all else being equal, EMCCDs will provide the highest SNR (Higher SNR is better). As light levels increase, sCMOS may be suitable, such as the cost-effective Thorlabs' Kiralux Compact Scientific CMOS cameras.
Experts vary on what features are the most important. And this is why it is important to decide ahead of time what type of imaging you’ll need and under what set of conditions. Prabala suggests generating a “hierarchy of values” to help navigate the many specifications. “This helps [scientists] allocate their research budget thoughtfully, ensuring that it is their application that drives the selection process.”
Says Mullan, “There are many specifications listed for cameras and this can make it difficult to work out what specifications actually matter to you, and the impact they may or may not have on your image quality. In addition, some of the key specifications may not be possible at the same time.” For example, adjusting for read noise, may impact speed. With that said, the three main parameters he considers to be of greatest general importance are: camera sensitivity, resolution, and speed.
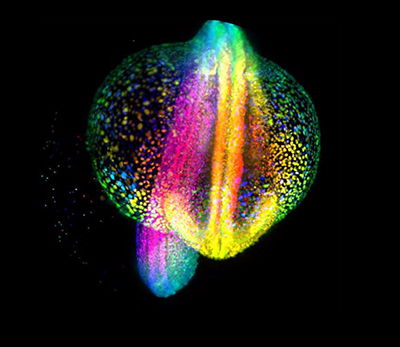
But do note, that you may also want to research dynamic range, shuttering mode, and accompanying software packages, or type of data analysis.
If you’re already feeling overwhelmed, don’t. The resources are endless. A simple place to start may be on a company’s website. Oftentimes, you can do side-by-side comparisons, such as with Olympus’ Camera Finder page.
Similarly, all of the specialists warn to never choose a camera without a demonstration using your own samples and optics. This is truly the only way to decide what camera will be the best solution for your needs. Ogama stresses that there is always a trade-off and that if you cannot prioritize the purpose of the camera now, then you may want to choose a multipurpose sCMOS.
Image: A developing zebrafish embryo imaged from 4 to 18 hours post fertilization where each cell nucleus is labeled with GFP. Cells are colorcoded for depth to visualize how dynamic cell reorganization gives rise to the body axis of zebrafish. Image courtesy of Gopi Shah, Max Planck Institute of Molecular Cell Biology and Genetics, Dresden.
In fact, the sCMOS came up repeatedly as a recommendation. While hesitant to make suggestions without fully understanding a lab’s requirements, Mullan says that for brightfield and fluorescent work, either exclusively, or a mix of imaging, an sCMOS may be the way to go, such as Andor’s Zyla 4.2 PLUS. For purely fluorescent imaging, he recommends considering either the Zyla or Andor’s new back-illuminated sCMOS, the Sona, for higher sensitivity and larger field of view.
For general documentation, Ogama recommends the DP74, a cooled CMOS camera. For customers who don’t need fluorescence, Olympus’ best sellers are the UC90 4K USB3.0 camera and the DP27 5 mega-pixel USB3.0 camera.
A final word of advice from Ogama: Any information lost with a mediocre microscope cannot be detected by any high-end camera. But on the other hand, even with top-of-the-line objectives, it doesn’t make sense to lose information with a low-end camera. “Balance is what microscope specialists and vendors do to help customers figure out the best solution.”
Hero image: NG108 cell line (hybridome with mouse (neuroblastoma) and rat (glioma)). Image courtesy of Olympus.